Contrast in cognition capacity between humans, macaques, and chimpanzees may relate to expression, rather than genetic, differences.
This webpage was produced for an undergraduate course at Davidson College.
In 2005, Hans Ellegren posed the question, “What does it take to make a human?” In response to the full sequencing of the genome of both humans and chimpanzees. In the years since, researchers have set about answering that question in a multitude of ways, but the difference that has most interested most of the world is the apparent difference in cognitive capacity. While repeated research has shown that chimpanzees have the capacity to solve puzzles, think through traps, and strategize solutions to complex social situations, there remain significant differences in the believed capacity of humans and chimpanzees (De Waal, 2016). Observational studies, like those in De Waal, test whether or not a chimpanzee, bonobo, macaque, or other animal can solve a puzzle- or learn to do so- by exposing these species to such tests (2016). They fail to look at the actual mechanics that determine this result. Genetics do not explain everything; an animal that learns how to solve a puzzle from a companion isn’t solving the puzzle because it is genetically predisposed to, but it is genetically predisposed to be able to learn from its companions.
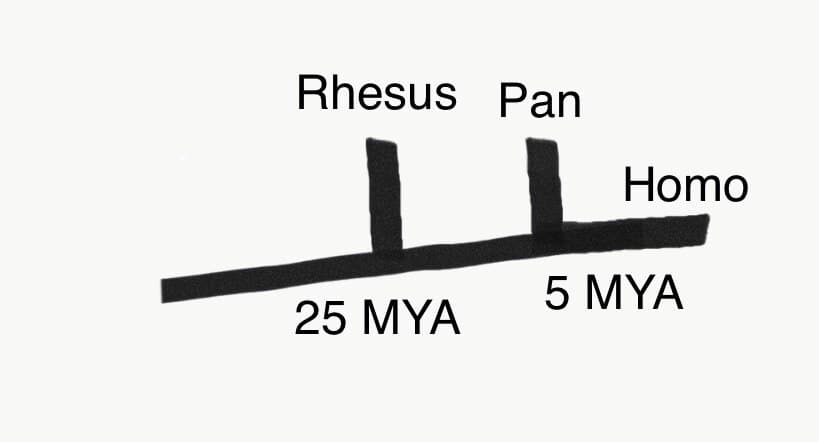
Kanton et. al. addressed this question by looking specifically at the developmental and adult differences in chimpanzees, macaques, and humans (2019). Much of their paper looked at differences in gene regulation and gene expression (Kanton et. al., 2019). Gene regulation, which will be abbreviated to regulation, are the mechanisms a cell uses to up-regulate (or increase the products, like proteins, of a gene), or down-regulate (or decrease/silence the products, like proteins, of a gene). Gene expression is a quantitative look at the results of regulation: how many proteins, with and without these regulators, are produced from this gene? The regulatory proteins and their resulting expression are combined in this paper to take a mechanistic look at potential differences in brain tissue development speed in chimpanzees, humans, and macaques.
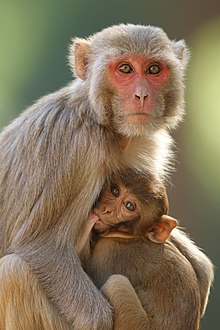
Stem cell development in the human brain is considerably slower than in chimpanzees and macaques, according to Kanton et. al. (2005). This “slow down” starts during the earliest stages of development and is, with a few exceptions, a difference relegated to development that isn’t found in adult tissues (Kanton et. al., 2019). It’s important to note that the difference in brain tissue development speed is not consistent across all human, chimpanzee, and macaque tissues. It isn’t just that humans have a greater in vivo development period than either of the other species studied. Human gestation is about 280 days, while chimpanzee gestation averages at 260 days, and rhesus macaques gestate for approximately 166 days (Encyclopedia Britannica). However, the overlap in timing between Homo and Pan gestation suggests that the approximately 40-week period predated the divergence in the species, indicating that the ratio of brain development to the rest of the tissues has likely shifted in humans as opposed to chimpanzees (DeSilva, 2011). Potentially accounting for that altered ratio, there are fundamental biological differences underlying the end of their gestation periods. Another study shows that, while great apes like chimpanzees and gorillas have brains that make up 10% of their body mass at birth, human brains are 12.3% of overall body mass at birth (DeSilva, 2011). While the relationship between brain-to-body ratio and brain development have not been formally correlated, this larger brain mass may prove interesting in determining the pressures that selected for slower brain development in humans than in other close relatives.
In addition to observing the development speed of brain tissues in the three species studied, Kanton et. al. found evidence for a slight difference in regulatory proteins between humans and chimpanzees and macaques (2005). This difference, for both enhancing and repressing proteins, was just under 10%, which, while a small percentage, is a significant difference in such closely related species (Kanton et. al., 2005). Development is guided and dictated not solely by genetic basis, but by regulation and interactions between genes and proteins. Understanding the differences in these factors helps contextualize the mechanisms for where evolution happened in human brain development. Evolution works with the materials that are already there; it cannot create the ideal system out of thin air. It’s also important to note the risks of mutations in the part of the genome that control development; anything that might change development, including its speed, could kill the individual and remove that trait from the population entirely. Changing a system, or developing one, has to be advantageous at every step of the way. Regulation is a spectrum– it isn’t all or nothing– and it can be adjusted to up-regulate, or down-regulate, across generations. Changing development by differentially regulating the mostly-conserved genes that account for development may have made these changes advantageous at every step of the way, rather than an all-or-nothing approach.
“What does it take to make a human?” (Ellegren, 2005). It may not be simply in which new genes exist in humans that don’t in close relatives; 98.8% of human and chimpanzee DNA is shared (AMNH). However, that isn’t to say that 98.8% of the development and gene regulation in humans and chimpanzees is shared. So much of life’s building blocks are conserved by sheer necessity, that life really isn’t all in the DNA. Human development is more complicated than which genes specifically make it possible and understanding how development works can help medical professionals better treat problems that arise during pregnancy. Additionally, understanding the normal cell growth rates and how cell growth rate may change can help doctors recognize abnormal growth rates and potentially develop treatments for rapidly growing tissues, like cancers. While taking each new genetic discovery and applying it to medical treatments is a critical function of genetic research, it’s also important to consider how results may shift our understanding of humanity as a species. Understanding the differences in our development and cognition from our closest relatives can help us understand the limits of our cognitive and empathetic abilities. Understanding how we relate to those close relatives, and how we’re different, helps to answer the question, which has plagued scientists, theologians, and philosophers for thousands of years: why are we different than other species on this planet, which do not have civilizations nor religions nor the written word?
References:
American Museum of Natural History (AMNH). “DNA: Comparing Humans and Chimps.” HAll of Human Origins, online.
DeSilva, Jeremy M. “A shift toward birthing relatively large infants early in human evolution.” Proceedings of the National Academy of Sciences 108.3 (2011): 1022-1027.
De Waal, Frans. Are We Smart Enough to Know How Smart Animals Are? New York, Norton and Company Inc. (2016).
Ellegren, Hans. “Evolution: natural selection in the evolution of humans and chimps.” Current biology 15.22 (2005): R919-R922.
Encyclopedia Britannica. “Primate- Natural History: Reproduction and Life Cycle.” Encyclopedia Britannica online.
Kanton, Sabina, et al. “Organoid single-cell genomic atlas uncovers human-specific features of brain development.” Nature 574.7778 (2019): 418-422.