This web page was produced as an assignment for an undergraduate course at Davidson College.
Sequencing ancient Roman genomes reveals two major ancestral shifts in Roman DNA: the first at the start of the Neolithic Age and the second prior to the Iron Age.
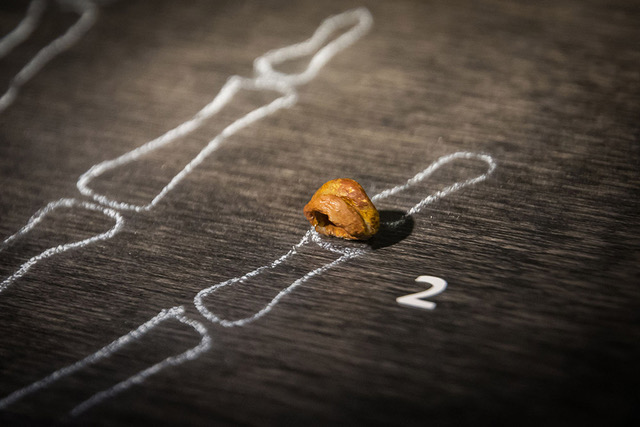
Many historians dedicate their lives to the study of Roman history, but not much is known about Roman genetics. Thankfully, technological advancements in 2008 helped reduce the cost of genome sequencing (Bentley et al. 2008). In the field of paleogenomics, the study of genomic information extracted from fossils, these sequencing advancements allowed for more cost efficient paleogenomics studies (Guimaraes et al. 2017). Not only does paleogenomics provide insight about diets and migratory paths, but it can also reveal ethnic ancestors and population interactions. By using paleogenomics, the researchers in “Ancient Rome: A genetic crossroads of Europe and the Mediterranean” looked to determine major ancestral shifts in Roman DNA during key time periods of Roman history (Antonio et al. 2019)
Initially, the researchers selected and sequenced 127 ancient individuals spanning Roman history. The researchers then identified three distinct genome compositions that corresponded with three different time periods in Roman history. The first group consisted of Mesolithic hunter-gatherers (10,000 to 7,000 BCE). The second was Neolithic and Copper Age individuals (7,000 to around 2,900 BCE), and the third was a group of individuals from the Iron Age to present day (900 BCE to date).
With the individuals organized into groups, the researchers focused on individual genomes in order to determine admixture and ancestry within each of the three groups. Admixture is the presence of DNA in a genome that came from a previously isolated population as a result of interbreeding.
In the Mesolithic Period, Romans were mainly hunter-gatherers, and they had low genetic diversity with minimal admixture. This is most likely due to the limited interactions between other populations of people on the European mainland. At the time, transportation was sparse and there was no demand for trade between populations. Shortly after the introduction of farming, however, the researchers detected a sharp increase in genetic diversity.
The researchers label the time period from 7,000-6,000 BCE as the “Neolithic Transition” because admixture analysis showed that individuals sequenced from the Neolithic Age had higher genetic diversity than individuals from the Mesolithic Period. Neolithic genomes had admixture from northwestern Anatolia farmers, Neolithic Iranian farmers, and Caucasus hunter-gatherers. Not only did this indicate interactions between population groups, but it also showed with whom the Romans interacted.
The second major ancestral shift took place around 2,900-900 BCE. The researchers know a large ancestral shift took place during this period because they sequenced individuals from the Iron Age (500-332 BCE) who had large portions of admixture not previously present in Neolithic Roman genomes. The date range for this second ancestral shift is wide because of the researcher’s limited sample size. None of the individuals sequenced were closer to the time of the ancestral shift, so it was difficult to identify when exactly this shift took place.
The individuals in the later Iron Age had admixture from Steppe-related people. The Steppe people lived further away from the Romans than any other ancestor that had previously contributed DNA to the Roman genome. This new admixture is most likely due to major technological developments in transportation that allowed people to travel further and faster.
Iron Age individuals also showed varied levels of ancestry from all around Europe, which might be as a result of migration into Roman territory during this time. Populations isolated from Romans during the Copper and Bronze Ages now interbred and contributed admixture to the Roman genome.
Once the researchers established these two main ancestral shifts, they then looked to understand why the Roman genome has unusually high diversity today. By examining the correlation between increased Roman power and increased admixture, the researchers inferred that as Rome expanded Roman citizens continued to increase their interactions with nearby nations. More interactions lead to more interbreeding and admixture.
During the Imperial Era, Rome was a central hub for trade and many nations used Rome’s elaborate trade routes to transfer goods around the Mediterranean. Genomic evidence supports these historiographical ideas, as we see a rise in admixture from various Mediterranean and North African ancestors in the time of Imperial Rome.
In the same way there was a positive correlation between increased influence and higher genetic diversity, the researchers noticed a similar correlation between decreased influence and lower genetic diversity. In Late Antiquity (284-700 CE) when Rome began to decline, the sequenced individuals showed a lower rate of heterozygosity which indicated lower genetic diversity. Less interaction and interbreeding resulted in less diversity.
While this paper focuses on the major ancestral shifts of Roman history, it only discusses the causes of the second ancestral shift (inflated Roman power). The researchers briefly mention that the first major shift was due to the development of farming, but how does farming lead to increased interactions? Some authors posit that the Neolithic Era was a time of migration and interbreeding (Zhang et al. 2016). Was this why Rome had more admixture during this period?
Also, the wheel was discovered around 3,500 BCE, but the researchers did not detect an ancestral shift then. It might be interesting for future researchers to sequence individuals from around 3,500 BCE to see if there are more subtle shifts in genetic diversity.
Walker A. Willis is currently enrolled in Davidson College. Contact him at wawillis@davidson.edu.
References
Antonio ML, Gao Z, Moots HM, Lucci M, Candilio F, Sawyer S, Oberreiter V, Calderon D, Devitofranceschi K, Aikens RC, et al. “Ancient Rome: A genetic crossroads of Europe and the Mediterranean.” Science. 2019 Nov 8;366(6466):708-714. doi: 10.1126/science.aay6826. PMID: 31699931; PMCID: PMC7093155.
Bentley DR, Balasubramanian S, Swerdlow HP, Smith GP, Milton J, Brown CG, Hall KP, Evers DJ, Barnes CL, Bignell HR, et al. “Accurate whole human genome sequencing using reversible terminator chemistry.” Nature. 2008 Nov 6;456(7218):53-9. doi: 10.1038/nature07517. PMID: 18987734; PMCID: PMC2581791.
Guimaraes S, Pruvost M, Daligault J, Stoetzel E, Bennett EA, Côté NM, Nicolas V, Lalis A, Denys C, Geigl EM, Grange T. “A cost-effective high-throughput metabarcoding approach powerful enough to genotype ~44 000 year-old rodent remains from Northern Africa.” Molecular Ecology Resources. 2017 May;17(3):405-417. doi: 10.1111/1755-0998.12565. Epub 2016 Jul 20. PMID: 27374145.
Zhang Y, Li J, Zhao Y, Wu X, Li H, Yao L, Zhu H, Zhou H. “Genetic diversity of two Neolithic populations provides evidence of farming expansions in North China.” Journal of Human Genetics. 2017 Feb;62(2):199-204. doi: 10.1038/jhg.2016.107. Epub 2016 Sep 1. PMID: 27581844.